HiLAT-RASM: Analyzing the Models that Simulate Polar Processes – Progress in 2020
A Core Project in DOE's Earth and Environmental System Modeling (EESM) Program Focuses on Analyzing Simulations of Conditions and Processes in the Arctic and the Southern Ocean
Environmental changes in the world’s polar regions are happening faster than anywhere else on the globe. Temperatures in the high latitudes are rising at a pace four times faster than the global mean. The outward signs of these changes are dramatic, including thawing permafrost on land and shrinking ice cover at sea. Such accelerating changes impact the climate and weather dynamics of the whole world.
To the U.S. Department of Energy (DOE), all this poses challenges and provides opportunities. Energy infrastructure in the Arctic is threatened, yet an ice-free Arctic Ocean may open speedy sea lanes for commercial shipping. Vast stores of methane in once permanently frozen ground are poised to respire into the atmosphere. Yet once frozen-over oceans are crowded with early blooms of phytoplankton, which may soak up more carbon dioxide.
Addressing the pressures and promise of arctic and antarctic changes requires simulations of likely conditions in the future. That’s the work of the DOE’s Earth and Environmental System Modeling (EESM) and the related Regional & Global Model Analysis (RGMA) program area. Within that realm resides the High-Latitude Application and Testing of Earth System Models (HiLAT) Science Focus Area, in collaboration with the Regional Arctic Systems Model (RASM) project.
The principal investigator for HiLAT-RASM is Wilbert Weijer, a 20-year physical oceanographer at Los Alamos National Laboratory in New Mexico. He is joined by 24 scientists at other national laboratories, universities, and research institutions.
HiLAT-RASM’s multidisciplinary researchers analyze earth system model simulations of the complex land, ocean, and atmospheric processes in the polar regions. They grapple with the interconnected realities of the mid-latitudes, where both storm systems and changing patterns of ocean circulation transfer heat to the poles. In turn, storm systems spurred by changes in the Arctic send outbreaks of cold air and pulses of snow to the lower latitudes.
Changes in high latitudes affect global circulation, in part as the terminus of an increasing level of heat transport through the oceans and atmosphere from the midlatitude regions, which receive more solar energy.
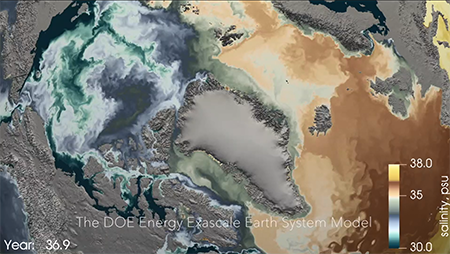
This coupled inter-latitudinal exchange of energy is influenced by the high-latitude processes that HiLAT-RASM investigators study. They explore four themes:
- How sea ice mediates the transport of heat in the oceans and atmosphere
- The role of these transport processes in arctic change
- The impacts of arctic changes in the rest of the world
- Predicting high-latitude environment change at the scale of decades
HiLAT-RASM scientists analyze models that simulate conditions in both the Arctic and in the Southern Oceans, in search of greater precision and predictive acuity. Models are always evolving and addressing biases and other deficiencies. They are both informed by observations and enhanced by them. After all, says PNNL earth scientist Hailong Wang, a HiLAT-RASM co-PI, “there are lots of inside processes that observations cannot give us.”
Some recent work:
AMOC: Ocean Circulation Distributes Heat
The Atlantic Meridional Overturning Circulation (AMOC) is an important component of the climate system. It plays a key role in the global redistribution of heat, as well as being a significant driver of carbon and heat sequestration in the subsurface ocean.

In a summary of recent work, Weijer writes: “The response of the AMOC to continued anthropogenic forcing will strongly determine the trajectory of Earth's climate in the 21st century and beyond.”
The HiLAT-RASM team investigates the drivers and impacts of AMOC, and its representation in earth system models. In Weijer et al. (2020), for instance, researchers compared the projected changes in the AMOC in the 21st century under models of different scenarios of socio-economic development.
Weijer also is the lead author of a 2019 paper on models predicting an AMOC decline in the 21st century, which reviews existing literature on the stability of the AMOC. None of the models appear to project a collapse of the AMOC in this century. Weijer says his team’s AMOC work will inform the Sixth Assessment Report by the Intergovernmental Panel on Climate Change, slated to appear in October 2021.
Shrinking snow and ice cover
The dramatic environmental changes in the Arctic include decreases in both terrestrial snow cover and sea ice. In Proceedings of the National Academy of Sciences paper, Zhang et al. (2019) found that these decreases account for about 70% of the satellite-observed reduction in arctic surface albedo since the 1980s.
What else contributes to this increase in observed arctic warming? A recent paper (Ren et al., 2020) found that global sulfate and soot changes during 2014-2018 contributed about 20%. At the same time, global and arctic cloud feedback is more uncertain and complicated than temperature and surface albedo feedbacks (Zhang et al., 2018 and Zhang et al., 2020.)

Estimating the surface albedo feedback―radiation reflected from the sea-ice surface―is central to modeling efforts. Donohoe et al. (2020) developed and introduced a new, simplified way to do it―one that revealed 40% more arctic albedo than previous estimates. Team members also contributed to a coordinated evaluation of regional climate model simulations using field campaign data from the 2014 Arctic Summer in Clouds Experiment (Sedlar et al. 2020). The study provides a road map for evaluating future models using data from field campaigns.
Heat Loss From Sudden Sea-Ice Holes
Changes in the Arctic are linked to changes in the exchange of heat between the ocean and atmosphere. In this regard, high-latitude oceans are critical regions―and part of that relates to polynyas. These naturally occurring holes in the winter sea ice pack can appear suddenly, exposing the atmosphere to the relatively warm ocean water and causing extreme ocean heat loss.
Polynyas in both the Arctic and Southern Oceans are the subject of HiLAT-RASM research. What triggers polynyas, investigators ask, and what impact do they have on the climate system?
To help answer these questions, the HiLAT-RASM team is investigating open-ocean polynyas in the Southern Ocean. One paper used the state-of-the-art climate model E3SMv0-HR to report on the causes and impacts of open-ocean polynyas (Kaufman et al., 2020). The authors found that polynya heat loss causes a persistent increase in surface air temperature over the Weddell Sea. In a companion study (Kurtakoti et al., 2020, in review), the HiLAT-RASM team investigated why small polynyas over Maud Rise (an oceanic plateau located in the Southern Ocean) sometimes transition to large Weddell Sea polynyas.

Critical Arctic River Deltas
Arctic rivers contribute a lot of fresh water, nutrients, sediments, and heat to the Arctic Ocean. That has a big effect on ocean salinity, temperature, and primary productivity. Riverine fluxes are modified by river deltas, which can drastically impact the location, timing, and magnitude of riverine fluxes to the Arctic Ocean. The effects of deltas are not represented in ESMs, which inspired Los Alamos National Laboratory earth scientist Anastasia Piliouras to improve the representation of arctic delta processes.
In one paper (Piliouras and Rowland, 2020), the authors characterized the morphologies of six major arctic deltas. Another paper (Vulis et al., 2020) shows how the seasonal dynamics of arctic delta lakes may be controlled by the structure of the channel network that influences localized permafrost thaw and subsurface flow.
The same flow routing method was used by Knights et al., 2020 to show how this algorithm can be used with biogeochemical models to identify hotspots of nitrate removal.
Another set of experiments showed that both ice and permafrost kept channel networks and the locations of riverine fluxes stable over long time periods. Researchers report on the first set of experiments, which separately examine the effects of ice and permafrost (Lauzon et al., 2019). In another paper, now in revision, researchers outline a second set of experiments (Piliouras et al.).
Modeling Arctic Marine Biogeochemistry
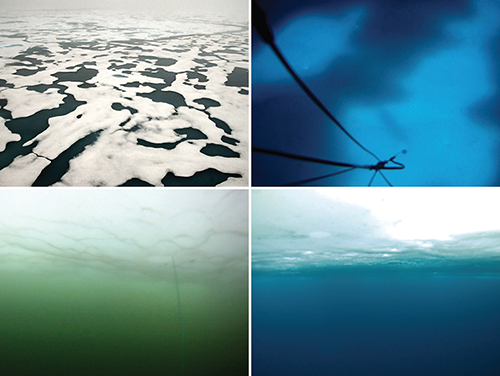
One dramatic sign of speeding environmental changes in the Arctic relate to increased phytoplankton growth and carbon fixation in the marginal seas of the Arctic Basin, which are exposed more and more to the sun. One study reported on massive phytoplankton blooms extending more than 100 kilometers (62 miles) under arctic sea ice (Arrigo et al. 2012). To date, such biological activity is poorly represented in models.
To correct that, in part, Naval Postgraduate School research professor Wieslaw Maslowski and others on the HiLAT-RASM team set out to improve models of arctic marine biogeochemistry. This effort led to an updated version of the CICE sea-ice model (Hecht et al., 2019) being deployed in the Energy Exascale Earth System Model (E3SMv0).
In related studies, researchers:
- Deployed a newer, improved version of the biogeochemical model E3SMv1.1-BGC to demonstrate up to an eight-fold underestimation of sea ice primary production of phytoplankton (Jeffery et al. 2020)
- Investigated the importance of model resolution and physics for reducing biases in marine biogeochemistry simulations (Jin et al. 2017)
- Used the regional configuration of RASM (Frants et al. 2020) to demonstrate the model’s skill in reproducing an under-sea-ice phytoplankton bloom (Arrigo et al. 2012)
- Showed an increase in under-sea-ice primary production over the last several decades (Clement Kinney et al. 2020)
To learn more about HiLAT-RASM, including program participants, fact sheets, research highlights, and publications, visit the project web page.
HILAT-RASM references
The Atlantic Meridional Overturning Circulation (AMOC)
- J Hirschi et al. (includes W Weijer), 2020. “The Atlantic meridional overturning circulation in high resolution models.” Journal of Geophysical Research - Oceans, 125, e2019JC015522. 10.1029/2019JC015522
- A Hu, L Van Roekel, W Weijer, OA Garuba, W Cheng, BT Nadiga. 2020. “Role of AMOC in transient climate response to greenhouse gas forcing in two coupled models.” Journal of Climate, 33, 5845-5859. 10.1175/JCLI-D-19-1027.1
- W Weijer, W Cheng, OA Garuba, A Hu, and BT Nadiga. 2020. “CMIP6 Models Predict Significant 21st Century Decline of the Atlantic Meridional Overturning Circulation.” Geophysical Research Letters, 47, e2019GL086075. 10.1029/2019GL086075
- W Weijer, W Cheng, SS Drijfhout, AV Fedorov, A Hu, LC Jackson, W Liu, EL McDonagh, JV Mecking, and J Zhang. 2019. “Stability of the Atlantic Meridional Overturning Circulation: A Review and Synthesis.” Journal of Geophysical Research: Oceans, 124, 10.1029/2019JC015083, 2019.
Arctic Clouds, Aerosol, Snow, Ice, and Radiative Processes
- Donohoe, A, E Blanchard-Wrigglesworth, A Schweiger, and PJ Rasch. 2020. “The Effect of Atmospheric Transmissivity on Model and Observational Estimates of the Sea Ice Albedo Feedback.” J. Climate, 33, 5743–5765, 10.1175/JCLI-D-19-0674.1
- Ren, L, Y Yang, H Wang, R Zhang, P Wang, and H Liao. 2020. “Source attribution of Arctic black carbon and sulfate aerosols and associated Arctic surface warming during 1980–2018.” Atmos. Chem. Phys., 20, 9067–9085, 10.5194/acp-20-9067-2020
- Sedlar, J, M Tjernstrom, A Rinke, A Orr, J Cassano, X Fettweis, X., et al. 2020. “Confronting Arctic troposphere, clouds, and surface energy budget representations in regional climate models with observations.” Journal of Geophysical Research: Atmospheres, 10.1029/2019JD031783
- Zhang, R., Wang, H., Fu, Q., Pendergrass, A. G., Wang, M., Yang, Y., Ma, P. L., and Rasch, P. J. 2018. "Local radiative feedbacks over the Arctic based on observed short‐term climate variations." Geophysical Research Letters, 45, 5761–5770, 10.1029/2019JD031783
- Zhang, R, H Wang, Q Fu, PJ Rasch, and X Wang, X. 2019. “Unraveling driving forces explaining significant reduction in satellite‐inferred Arctic surface albedo since the 1980s.” Proceedings of the National Academy of Sciences of the United States of America, 116(48), 23,947–23,953, 10.1073/pnas.1915258116
- Zhang, R, H Wang, Q Fu, and PJ Rasch. 2020. “Assessing global and local radiative feedbacks based on AGCM simulations for 1980–2014/2017.” Geophysical Research Letters, 47, e2020GL088063. 10.1029/2020GL088063
Polynyas
- Kaufman, ZS, N Feldl, W Weijer, and M Veneziani. 2020. “Causal Interactions between Southern Ocean Polynyas and High-Latitude Atmosphere-Ocean Variability.” Journal of Climate 33, pp. 4891-4905. 10.1175/JCLI-D-19-0525.1
- Kurtakoti, PK, M Veneziani, A Stöessel, W Weijer, and M Maltrud. 2020. “On the Generation of Weddell Sea Polynyas in a High-Resolution Earth System Model. Journal of Climate.” In revision.
- Lee, Y, W Maslowski, J Cassano, J Stroeve, R Osinski, S. Kamal. 2020a. “On the Evolution of Winter Polynyas Along Northern Greenland.” In preparation.
- Lee, Y, W Maslowski, R, Osinski, J Cassano, J Clement Kinney, A Craig, and M. Seefeldt. 2020b. “The evolution of summer polynyas along northern Greenland.” In preparation.
Terrestrial
- Piliouras, A, R Lauzon, and JC Rowland. “Unraveling the combined influence of ice and permafrost on delta morphodynamics.” Journal of Geophysical Research – Earth Surface. In Revision.
- Knights, D, A Sawyer, R Barnes, A Piliouras, J Schwenk, DA Edmonds, and A Brown. 2020. “Nitrate removal across ecogeomorphic zones in Wax Lake Delta, Louisiana (USA).” Water Resources Research. 10.1029/2019WR026867
- Vulis, L, A Tejedor, J Schwenk, A Piliouras, JC Rowland, and E Foufoula-Georgiou. 2020. “Channel network control on seasonal lake area dynamics on Arctic deltas.” Geophysical Research Letters. 10.1029/2019GL086710
- Schwenk, J, A Piliouras, and JC Rowland. 2020. “Determining flow directions in river channel networks using planform morphology and topology.” Earth Surface Dynamics. 10.5194/esurf-8-87-2020
- Piliouras, A and JC Rowland. 2020. “Arctic river delta morphologic variability and implications for riverine fluxes to the coast.” Journal of Geophysical Research – Earth Surface. 10.1029/2019JF005250
- Lauzon, R., A. Piliouras, and J. C. Rowland. (2019). Ice and permafrost effects on delta morphology and channel dynamics. Geophysical Research Letters. 10.1029/2019GL082792
Arctic Marine Biogeochemistry
- Clement Kinney, J, W Maslowski, R Osinski, M Jin, M Frants, N Jeffery, and YJ Lee. 2020. “Hidden production: On the importance of pelagic phytoplankton blooms beneath Arctic Sea ice.” Journal of Geophysical Research: Oceans, 125, e2020JC016211. 10.1029/2020JC016211
- Gibson, G, W Weijer, N Jeffery, and S Wang. 2020. “Relative impact of sea ice and temperature changes on Arctic marine production.” Journal of Geophysical Research: Biogeosciences, 125, e2019JG005343. 10.1029/2019JG005343
- Frants, M, W Maslowski, R Osinski, N Jeffery, M Jin, and J Clement Kinney. 2020. Evaluation of under Sea‐Ice Phytoplankton Blooms in the Fully‐Coupled, High‐Resolution Regional Arctic System Model (RASM), Preprint. ESSOAr. 10.1002/essoar.10503749.1
- Hecht, M, M Veneziani, W Weijer, B Kravitz, S Burrows, D Comeau, et al. 2019. “E3SMv0‐HiLAT: A modified climate system model targeted for the study of high‐latitude processes.” Journal of Advances in Modeling Earth Systems, 11, 2814–2843. 10.1029/2018MS001524
- Jeffery, N, M Maltrud, E Hunke, S Wang, J Wolfe, A Turner, S Burrows, X Shi, WH Lipscomb, W Maslowski, and K Calvin. 2020. “Investigating controls on sea ice algal production using E3SMv1.1‐BGC.” Annals of Glaciology, 1– 22. 10.1017/aog.2020.7
- Jin, M, C Deal, W Maslowski, P Matrai, A Roberts, R Osinski, YJ Lee, M Frants, S Elliott, N Jeffery, E Hunke, and S Wang. 2018. “Effects of model resolution and ocean mixing on forced ice‐ocean physical and biogeochemical simulations using global and regional system models.” Journal of Geophysical Research: Oceans, 123, 358– 377. 10.1002/2017JC013365